2025 №04 (02) |
DOI of Article 10.37434/tpwj2025.04.03 |
2025 №04 (04) |
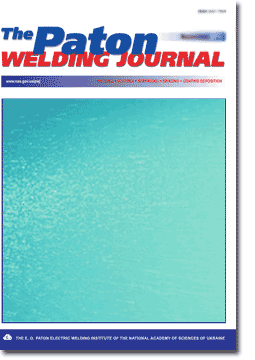
The Paton Welding Journal, 2025, #4, 12-21 pages
Application of microplasma deposition for 3D printing of aerospace engine parts
V.Yu. Khaskin1, K.M. Sukhyi2, O.V. Ovchynnykov1, O.V. Zaichuk2
1E.O. Paton Electric Welding Institute of the NASU. 11 Kazymyr Malevych Str., 03150, Kyiv, Ukraine. E-mail: khaskin1969@gmail.com2Ukrainian State University of Science and Technologies 2 Lazaryana Str., 49010, Dnipro, Ukraine
Abstract
The work is devoted to establishing the basic technological regularities and features of the formation of characteristic structures of metal layers during additive microplasma deposition with powders of corrosion- and heat-resistant alloys and determining the prospects of this process for 3D printing of aircraft parts. The work established that the choice of the mode of additive microplasma deposition of the selected group of powders is mainly determined by the size of the filler powder fraction. The energy input and thermal power of the constricted arc for growing metal products with a wall thickness of up to 3 mm using powders based on Fe and Ni with a fraction of 40‒100 μm were determined. The main features of the structure formation of the metal of samples produced by microplasma deposition, and their mechanical characteristics were determined, and the tendency to burnout of alloying elements of the deposited alloy was assessed. It is shown that despite the need for finishing mechanical treatment of critical functional surfaces, the use of microplasma deposition can be considered a fairly promising direction for 3D printing of metal parts of aircraft equipment.
Keywords: 3D printing, nickel alloy, microplasma deposition, metal powders, technological modes, dendritic structure, heat dissipation, mechanical properties
Received: 05.03.2025
Received in revised form: 24.03.2025
Accepted: 08.05.2025
References
1. Moon, S.K., Tan, Y.E., Hwang, J., Yoon, Y.-J. (2014) Application of 3D printing technology for designing light-weight unmanned aerial vehicle wing structures. Inter. J. of Precision Eng. and Manufacturing-Green Technology, 1, 223–228. DOI: https://doi.org/10.1007/s40684-014-0028-x2. Chee, K.C., Kah, F.L., Chu, S.L. (2010) Rapid prototyping: Principles and applications. 3rd Ed. World Scientific Publ. Co Pte Ltd. DOI: https://doi.org/10.1142/6665
3. Martinez, D.W., Espino, M.T., Cascolan, H.M. et al. (2022) A comprehensive review on the application of 3D printing in the aerospace industry. Key Eng. Materials, 913, 27–34. DOI: https://doi.org/10.4028/p-94a9zb
4. Singamneni, S., Lv, Y., Hewitt, A. et al. (2019) Additive manufacturing for the aircraft industry: A review. J. of Aeronautics & Aerospace Eng., 8(1), 214. DOI: https://doi.org/10.35248/2168-9792.19.8.215
5. Gadagi, B., Lekurwale, R. (2021) A review on advances in 3D metal printing. Materials Today: Proceedings, 45(1), 277–283. DOI: https://doi.org/10.1016/j.matpr.2020.10.436
6. Matthews, N. (2018) Chapter fifteen — Additive metal technologies for aerospace sustainment. Aircraft Sustainment and Repair, 2018, 845–862. DOI: https://doi.org/10.1016/B978-0-08-100540-8.00015-7
7. Gisario, A., Kazarian, M., Martina, F., Mehrpouya, M. (2019) Metal additive manufacturing in the commercial aviation industry: A review. J. of Manufacturing Systems, 53, 124–149. DOI: https://doi.org/10.1016/j.jmsy.2019.08.005
8. Yamazaki, T. (2016) Development of a hybrid multi-tasking machine tool: Integration of additive manufacturing technology with CNC machining. Procedia CIRP, 42, 81–86. DOI: https://doi.org/10.1016/j.procir.2016.02.193
9. Peleshenko, S., Korzhyk, V., Voitenko, O. et al. (2017) Analysis of the current state of additive welding technologies for manufacturing volume metallic products (Review). Eastern-European J. of Enterprise Technologies, 3–1(87), 42–52. DOI: https://doi.org/10.15587/1729-4061.2017.99666
10. Kumar, P., Jain, N.K. (2020) Effect of material form on deposition characteristics in micro-plasma transferred arc additive manufacturing process. CIRP J. of Manufacturing Sci. and Technol., 30, 195–205. DOI: https://doi.org/10.1016/j.cirpj.2020.05.008
11. Wang, H., Jiang, W.H., Valant, M., Kovacevic, R. (2003) Microplasma powder deposition as a new solid freeform fabrication process. Proc. of the Institution of Mechanical Eng., Pt B: J. of Eng. Manufacture, 217(12), 1641–1650. DOI: https://doi.org/10.1243/095440503772680578
12. Ovchinnikov, O.V., Duryagina, Z.A., Romanova, T.E. et al. (2021) Powder titanium alloys for additive technologies: Structure, properties, modeling. Kyiv, Naukova Dumka [in Ukrainian].
13. Rezydent, N., Stepanova, N. (2023) Using the solid works flow simulation CFD package to study the performance indicators of the cyclone-utilizer. Modern Technology Materials and Design in Construction, 33(2), 192–197. DOI: https://doi.org/10.31649/2311-1429-2022-2-192-197
14. Akca, E., Trgo, E. (2015) Metallographic procedures and analysis — A review. Periodicals of Eng. and Natural Sci. (PEN), 3(2), 9–11. DOI: https://doi.org/10.21533/pen.v3i2.51
15. Korzhyk, V., Gao, S., Khaskin, V. et al. (2024) Features of the stress-strain state of 3D metal objects produced by additive microplasma deposition of the powder of a Fe–Cr–Ni–B–Si system. Applied Sci., 14, 4159. DOI: https://doi.org/10.3390/app14104159
16. Sidharth, Rana R., Pandey S. (2023) Configuring microplasma for material process optimization. Materials Today: Proceedings. DOI: https://doi.org/10.1016/j.matpr.2023.01.339