2021 №01 (04) |
DOI of Article 10.37434/sem2021.01.05 |
2021 №01 (06) |
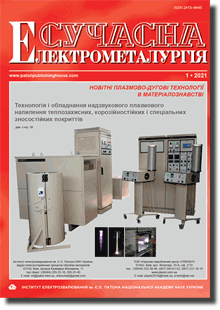
Сучасна електрометалургія, 2021, #1, 39-47 pages
Мікроструктура та механічні властивості деталей із високоміцних титанових сплавів, отриманих методом WAAM (Огляд)
Р.В. Селін, С.Л. Шваб, М.М. Димань
ІЕЗ ім. Є.О. Патона НАН України. 03150, м. Київ, вул. Казимира Малевича, 11. E-mail: office@paton.kiev.ua
Реферат
Адитивне виробництво (Additive Manufacturing) — це створення виробів, засноване на поетапному додаванні матеріалу на основу у вигляді плоскої платформи або осьової заготовки. Wire Arc Additive Manufacturing дуже перспективна технологія, що дозволяє виготовляти великогабаритні деталі складної форми з матеріалів з високою доданою вартістю. Ця технологія є частиною процесів адитивного виробництва, яка використовує металеві дроти в якості присадного матеріалу, а дугу, як джерело енергії. В даній оглядовій роботі розглянуто процес отримання деталей із титанових сплавів методом Wire Arc Additive Manufacturing, який має суттєві переваги перед іншими процесами адитивного виробництва — високі ефективність використання ресурсів та продуктивність, низьку вартість обладнання. Отримання деталей із високоміцних титанових сплавів за допомогою методу Wire Arc Additive Manufacturing дозволяє контролювати мікроструктуру титанових сплавів, що необхідно, оскільки високоміцні титанові сплави дуже чутливі до термічного циклу. Розглянуто різні методи контролю мікроструктури деталей із титанових сплавів, її властивості та вплив на механічні показники деталі. Бібліогр. 39, табл. 3, рис. 9.
Ключові слова: адитивне виробництво; WAAM; високоміцні титанові сплави; мікроструктура; механічні властивості
Received 22.01.2021
Список літератури
1. Martina, F., Colegrove, P.A., Williams, S.W., Meyer, J. (2015) Microstructure of interpass rolled wire+arc additive manufacturing Ti–6Al–4V components. Metallurg. and Mater. Transact. A, 46(12), 6103–6118.2. Bekker, A.C., Verlinden, J.C. (2018) Life cycle assessment of wire+ arc additive manufacturing compared to green sand casting and CNC milling in stainless steel. J. of Cleaner Production, 177, 438–447.
3. Lütjering, G.,Williams, J.C. (2007) Titanium. Springer Science & Business Media.
4. Wang, Q., Ji, C., Wang, Y. et al. (2013) β-Ti alloys with low young’s moduli interpreted by cluster-plus-glue-atom model. Metallurg. and Mater. Transact. A, 44(4), 1872–1879.
5. DebRoy, T., Wei, H.L., Zuback, J.S. et al. (2018) Additive manufacturing of metallic components–process, structure and properties. Progress in Materials Sci., 92, 112–224.
6. Wang, F., Williams, S., Rush, M. (2011) Morphology investigation on direct current pulsed gas tungsten arc welded additive layer manufactured Ti6Al4V alloy. Inter. J. Adv. Manuf. Technol., 57(5), 597–603.
7. Ding, D., Pan, Z., Cuiuri, D. et al. (2016) Bead modelling and implementation of adaptive MAT path in wire and arc additive manufacturing. Robotics and Computer-Integrated Manufacturing, 39, 32–42.
8. Wang, F., Williams, S., Colegrove, P., Antonysamy, A.A. (2013) Microstructure and mechanical properties of wire and arc additive manufactured Ti–6Al–4V. Metallurg. and Mater. Transact. A, 44(2), 968–977.
9. Mereddy, S., Bermingham, M.J., Kent, D. et al. (2018) Trace carbon addition to refine microstructure and enhance properties of additive-manufactured Ti–6Al–4V. JOM, 70(9), 1670–1676.
10. Mereddy, S., Bermingham, M.J., StJohn, D.H., Dargusch, M.S. (2017). Grain refinement of wire arc additively manufactured titanium by the addition of silicon. J. of Alloys and Compounds, 695, 2097–2103.
11. Zuback, J.S., Palmer, T.A., DebRoy, T. (2019) Additive manufacturing of functionally graded transition joints between ferritic and austenitic alloys. Ibid., 770, 995–1003.
12. Onuike, B., Bandyopadhyay, A. (2018) Additive manufacturing of Inconel 718 Ti–6Al–4V bimetallic structures. Additive Manufacturing, 22, 844–851.
13. Wang, J., Pan, Z., Ma, Y. et al. (2018) Characterization of wire arc additively manufactured titanium aluminide functionally graded material: microstructure, mechanical properties and oxidation behaviour. Mater. Sci. and Engin. A, 734, 110–119.
14. Hernández-Nava, E., Mahoney, P., Smith, C.J. et al. (2019) Additive manufacturing titanium components with isotropic or graded properties by hybrid electron beam melting/hot isostatic pressing powder processing. Sci. Reports, 9(1), 1–11.
15. Baufeld, B., Brandl, E., Van der Biest, O. (2011) Wire based additive layer manufacturing: Comparison of microstructure and mechanical properties of Ti–6Al–4V components fabricated by laser-beam deposition and shaped metal deposition. J. Materials Proc. Technology, 211(6), 1146–1158.
16. Åkerfeldt, P., Antti, M.L., Pederson, R. (2016) Influence of microstructure on mechanical properties of laser metal wire-deposited Ti–6Al–4V. Mater. Sci. and Engin. A, 674, 428–437.
17. Donoghue, J., Antonysamy, A.A., Martina, F. et al. (2016) The effectiveness of combining rolling deformation with Wire– Arc Additive Manufacture on β-grain refinement and texture modification in Ti–6Al–4V. Materials Characterization, 114, 103–114.
18. Antonysamy, A.A., Meyer, J., Prangnell, P.B. (2013) Effect of build geometry on the β-grain structure and texture in additive manufacture of Ti–6Al–4V by selective electron beam melting. Ibid., 84, 53–168.
19. Antonysamy, A.A., Prangnell, P.B., Meyer, J. (2012) Effect of wall thickness transitions on texture and grain structure in additive layer manufacture (ALM) of Ti–6Al–4V. Mat. Sci. Forum, 706, 205–210. Transact. Tech. Publ. Ltd.
20. Al-Bermani, S.S., Blackmore, M.L., Zhang, W., Todd, I. (2010) The origin of microstructural diversity, texture, and mechanical properties in electron beam melted Ti–6Al–4V. Metallurg. and Mater. Transact. A, 41(13), 3422–3434.
21. Vilaro, T., Colin, C., Bartout, J.D. (2011) As-fabricated and heat-treated microstructures of the Ti–6Al–4V alloy processed by selective laser melting. Ibid., 42(10), 3190–3199.
22. Bantounas, I., Dye, D., Lindley, T.C. (2010) The role of microtexture on the faceted fracture morphology in Ti–6Al–4V subjected to high-cycle fatigue. Acta Materialia, 58(11), 3908–3918.
23. Ho, A., Zhao, H., Fellowes, J.W. et al. (2019) On the origin of microstructural banding in Ti–6Al–4V wire-arc based high deposition rate additive manufacturing. Ibid., 166, 306–323.
24. Alonso, U., Veiga, F., Suárez, A., Artaza, T. (2020) Experimental investigation of the influence of wire arc additive manufacturing on the machinability of titanium parts. Metals, 10(1).
25. Cong, B., Ding, J., Williams, S. (2015) Effect of arc mode in cold metal transfer process on porosity of additively manufactured Al–6.3 % Cu alloy. The Inter. J. Adv. Manuf. Technol., 76(9‒12), 1593–1606.
26. Ou, W., Mukherjee, T., Knapp, G.L. et al. (2018) Fusion zone geometries, cooling rates and solidification parameters during wire arc additive manufacturing. Inter. J. of Heat and Mass Transfer, 127, 1084–1094.
27. Ding, D., Wu, B., Pan, Z. et al. (2020) Wire arc additive manufacturing of Ti–6Al–4V using active interpass cooling. Materials and Manufacturing Processes, 35(7), 845–851.
28. Zhou, Y., Qin, G., Li, L. et al. (2020) Formability, microstructure and mechanical properties of Ti–6Al–4V deposited by wire and arc additive manufacturing with different deposition paths. Mater. Sci. and Engin. A, 772, 138654.
29. Wu, B., Pan, Z., Ding, D. et al. (2018) The effects of forced interpass cooling on the material properties of wire arc additively manufactured Ti–6Al–4V alloy. J. of Materials Proc. Technology, 258, 97–105.
30. Davis, A.E., Breheny, C.I., Fellowes, J. et al. (2019) Mechanical performance and microstructural characterisation of titanium alloy-alloy composites built by wire-arc additive manufacture. Mater. Sci. and Engin. A, 765, 138289.
31. (2013) ASTM Standard E399: Standard test method for linear-elastic plane-strain fracture toughness KIС of metallic materials. ASTM Inter., Pennsylvania, US.
32. Attar, H., Ehtemam-Haghighi, S., Kent, D., Dargusch, M.S. (2018) Recent developments and opportunities in additive manufacturing of titanium-based matrix composites: A review. Inter. J. of Machine Tools and Manufacture, 133, 85–102.
33. Martina, F., Williams, S.W., Colegrove, P.A. (2014) Improved microstructure and increased mechanical properties of additive manufacture produced Ti–6Al–4V by interpass cold rolling. In: Proc. of 24th Inter. Solid Freeform Fabrication Symp. (12–14 August, 2014, TX, USA), 490–496.
34. Wang, F., Williams, S., Colegrove, P., Antonysamy, A.A. (2013) Microstructure and mechanical properties of wire and arc additive manufactured Ti–6Al–4V. Metallurg. and Mater. Transact. A, 44(2), 968–977.
35. Bantounas, I., Dye, D., Lindley, T.C. (2010) The role of microtexture on the faceted fracture morphology in Ti–6Al–4V subjected to high-cycle fatigue. Acta Materialia, 58(11), 3908–3918.
36. Smirnov, I., Polyakov, A., Sudenkov, Y. (2017) Strength and fracture of ultrafine-grained titanium Grade 4. Procedia Structural Integrity, 6, 196–200.
37. Attar, H., Calin, M., Zhang, L.C. et al. (2014) Manufacture by selective laser melting and mechanical behavior of commercially pure titanium. Mater. Sci. and Engin. A, 593, 170–177.
38. Yamanaka, K., Saito, W., Mori, M. et al. (2015) Preparation of weak-textured commercially pure titanium by electron beam melting. Additive Manufacturing, 8, 105–109.
39. (1994) ASM International, Materials Properties Handbook: Titanium Alloys. Materials Park, OH.